What to Expect:
In this episode, we explore Ketan Madane’s innovative research on fluidic devices that enhance chemical processes. Ketan’s work aims to develop processes that are modular, agile, intensified, and continuous (MAGIC), which improve efficiency and effectiveness in chemical engineering.
About the Guest:
Ketan Madane
Ketan Madane is a PhD student at the University of Limerick, Ireland. He holds a Bachelor’s degree in Mechanical Engineering from India and has experience in teaching and industry roles. Ketan’s research aims to optimize chemical processes using fluidic devices, combining computational fluid dynamics with experimental techniques.
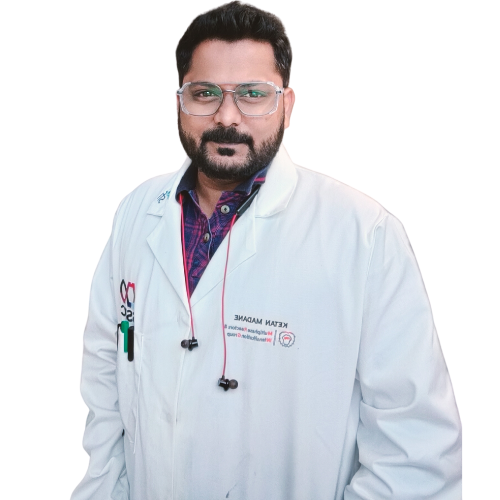
🌟 Key Takeaways from This Episode:
- MAGIC Processes: Ketan’s research focuses on developing processes that are modular, agile, intensified, and continuous (MAGIC), enhancing the efficiency and effectiveness of chemical engineering processes.
- Career Path: Ketan’s journey from mechanical engineering in India to a PhD in fluidic devices at the University of Limerick illustrates his dedication and expertise in the field.
- Favorite Experiment: Ketan’s favorite experiment involves using fluidic oscillators and vortex diodes to enhance chemical processes, particularly in the crystallization of paracetamol, leading to reduced particle size and improved process efficiency.
🔬 In This Episode, We Cover:
Ketan’s Research
Ketan’s research focuses on designing and developing fluidic devices to improve chemical processes. These devices, which are modular, agile, intensified, and continuous (MAGIC), help in making chemical reactions more efficient and effective. He uses computational fluid dynamics and experimental techniques to optimize these devices.
Ketan’s Career Journey
Ketan started his career with a Bachelor’s in Mechanical Engineering in India. He worked in the manufacturing industry and academia before pursuing a PhD at the University of Limerick. His journey reflects a strong commitment to advancing chemical engineering through innovative research.
Ketan’s Favourite Research Experiment
One of Ketan’s proudest achievements is enhancing the anti-solvent crystallization of paracetamol using fluidic oscillators and vortex diodes. This innovative approach significantly reduced particle size and improved the efficiency of the crystallization process.
Life as a Scientist: Beyond the Lab
Ketan enjoys the endless curiosity and pursuit of knowledge that come with being a scientist. He values the collaborative nature of academia, which allows for the exchange of ideas and collective problem-solving.
Ketan’s 3 Wishes
- Bridging mechanisms between interdisciplinary fields: Ketan wishes for better integration and collaboration across different scientific disciplines.
- Unlimited access to cutting-edge technology and computational tools: He advocates for unrestricted access to advanced experimental and computational resources.
- Sustainable research funding and recognition: Ketan emphasizes the need for stable funding and proper recognition of scientific contributions.
Ketan’s Time on @RealSci_Nano
Ketan will be showcasing various fluidic devices, their applications, and simulations on the RealSci_Nano Twitter account. Followers can expect to see the importance of fluid dynamics in chemical engineering and the innovative ways these devices are used to improve industrial processes.
Join the Conversation
Follow & listen to “Under the Microscope” on Spotify, Apple Podcasts, Castbox, Amazon Music, Goodpods.
Got your own favourite podcast app? Follow the RSS link to find Under the Microscope.
Watch the video of all Under the Microscope episodes on The Science Talk’s YouTube channel. While you are at it, make sure to subscribe to our YouTube Channel.
Learn more about Under the Microscope – https://thesciencetalk.com/under-the-microscope/
Love what we do? Support us:
Be our Patron – https://www.patreon.com/TheScienceTalk
We also offer partnerships to scientists, research institutions, industry, funding bodies & societies.
Get in touch to start the conversation.
Transcript
[00:00:00] Hi there, and welcome to Under the Microscope, your podcast spotlighting materials and nanoscience. My name is Svenja Lohmann. I’m your host for today. And with me, I have again, a guest who is a scientist. And his name is And he’s a PhD student working at the University of Limerick in Ireland. Welcome. How are you today?
I’m very good. Thank you very much. First of all, thank you very much for having me. I feel privileged and honored to be on your podcast. Thank you very much for giving me this opportunity. Thank you for joining us and sharing your science and scientific journey with us. We are, I think, very exciting for your research and your research topic.
I think it’s good. Different, maybe, uh, for me at least, I think I, I will learn a lot, so let’s maybe start straight away. So can you explain your current research to us in very simple words, please? [00:01:00] Yeah. So in very simple words, what I do is we create magic processes. So, so magic essentially stands for, um, we develop processes that are modular, which are agile, uh, which are intensified and which are continuous.
So that stands for magic. Any process that we do in today’s industry is batch process. So what you do is that you have a simple stirred tank reactor. Uh, you have a component A into that, and then you add component B. So that is a batch process. So, but that batch process suffers so many disadvantages. So we need to make this continuous process.
So essentially if there is a process that is there, we add the magic into it and we can, uh, magic processes. So that’s what we do. And my research is. Focused on designing and developing, uh, fluidic devices to facilitate this magic, uh, processes. Okay. Yeah. I just stopped you quickly right there. Um, maybe you were going to say it anyway, then sorry for the interruption.
Can you just give a very simple explanation of a fluidic device? Because I’m not sure if all the listeners know what it is. Simplest fluidic device that we [00:02:00] have in any laboratory or we use it is a simple pipe. Okay. That was simple. That is very simple, right? That is the simplest fluidic device that we work on.
And most of the labs across the world and all the laboratories, we use a T junction, right? We have a simple pipe, and then we have a T junction, a component A is going on one inlet, and the second component meets at a T junction. And then that whatever reaction or whatever processes is taking place that will take place at the junction or maybe down the timeline down the downstream of the pipe and the process completes.
So that’s a simple fluidic device. Okay. Thank you. This is a simple fluidic device or rather, you know, there is another device that I want to show you that this is a helical coil, a simple pipe, which is wrapped helically around an axis. For those listening on Spotify, Arthur podcast or any other just audio, he actually brought some devices.
So you might want to head over to the YouTube channel so you can actually see these devices. Yes. So this again, as I was saying that, you know, so now what we have done is that we have converted a straight pipe into a helical coil, right? This is a helical coil. So now what happens is basically once you change [00:03:00] this heli, uh, straight pipe into a helical coil, the entire fluid dynamics inside the device changes.
And then you have a better performance in terms of let’s say mixing or residence time or residence time distribution. So again, now this was, we were talking about pipes. So there is another version of a pipe CFI coil flow inverter. We call this coil flow inverter. So we have helical coil at one section.
Then it goes to another section, which is 90 degree. Then it goes to another section, which is again, 90 degree. So this is a coil flow inverter. Okay. So it looks like a Cross of, uh, helical coils or turbos. Yes, correct. So again, once you go from straight pipe to helical coil to coil flow inverter, fluid mechanics entirely changes.
The performance of a straight pipe is less, uh, the helical coil performs better, uh, in a certain sense. And the helical coil, coil flow inverter, uh, actually works better, uh, than the helical coil. So now these are simple fluidic devices to understand. Mm-Hmm. . There are numerous fluidic devices and there is, there is ample amount of literature available on all the D flu devices that are being used for different processes.
You have static [00:04:00] mixers, you have microfluidics. These are also fluidic devices. But my pH is focused on two devices. One is fluidic oscillator, and uh, second is vortex dialed. So fluidic oscillator, as the name suggests, it actually oscillates a jet of a fluid. So the interesting thing is that the fluidic oscillator actually was never invented for the fact to be used for process engineering applications.
So the fluidic oscillator is a device that was invented in 1960s. And, uh, it was the, the replacement of then MROIC, MROIC transistors. So basically in 1960s, people are actually investing a lot of time and energy to generate fluidic computers. Okay. So like, uh, I guess not water based, but some kind of liquid instead of a silicon chip?
Yes, correct. So after After the invention of p n junction diode and all the semiconductor technology, this field was a little bit, um, I mean, of course, it was replaced by, uh, the electronic transistors, because it is very simple to, even if you use a simple pipe, uh, to, uh, connections of pipe here and there, you can still construct a logic gate out of it, but of course, you know, these devices are bulky, and [00:05:00] eventually p n junction replaced it, but this device was there, and this device actually holds a beautiful property, it oscillates the jet, of a fluid, and why not we harness this oscillatory mechanism for process engineering application?
That was the question that we asked ourselves. What we do essentially is that we study all these devices for transport phenomena, which essentially means we quantify mixing in them, we quantify residence time distribution in them, we quantifies to some extent, sometimes heat transfer and mixing. Now, all the processes, chemical processes, or all the process engineering applications, uh, involves one important thing that is mixing.
So, If we have a device, we quantify these transport phenomena in these devices and then use these devices for real time applications, real applications. So as I said, that fluidic oscillator is something, uh, as name suggests, there is a jet of fluid that enters a confined space and it just starts oscillating by the virtue of geometry.
Okay, so there is no. No, no. No [00:06:00] motor or something or motor is rubber. But you know, there’s no part that oscillates the fluid. It’s just the design of the device. Yes. So essentially the main part of my PHD is I am working in devices without moving parts. Okay. So you basically, you just have a device and just by shaping it in a very specific way, Okay.
You influence the fluid dynamics. So it does whatever you want to do. Yes. So how to just because of shape, it doesn’t happen. Sometimes very interesting fluid dynamics is always at place. Now, uh, Henry Konda is one of the Romanian, uh, scientists. He invented a physical phenomenon, fluid dynamic phenomena called as Konda effect.
That effect is known after his name. So what he did was I can give you a simple example. Suppose you are actually watering your plants in garden and you are holding a hose pipe, right? And if you try to bring your finger near the jet of that water jet, that jet tries to stick to your thumb. Right? So that is [00:07:00] called as Coanda effect.
Okay. Right. And how to harness this effect now, right? So how to harness this effect. So what happens essentially in Coanda effect is that if there is a jet that is coming, what happens is that jet tries to entrain fluid, the surrounding fluid inside it. Because it is going in this direction, it tries to enter in surrounding fluid inside inside the jet.
Yeah. If you bring a wall near it, that entrainment is restricted. Hence the jet to balance the momentum, it gets attached itself to the wall. Okay. Yeah. And that effect is called as Quanda effect. And fluidic oscillators works on Quanda effect. Okay. Interesting. So you built something that’s, I guess this is slightly a bit more complicated than Uh, watering holes for a garden, but we see some similarities here.
Okay. I do have a device to show you. So this is a fluidic oscillator. Okay, so which part exactly is the oscillator? So basically, you have a cavity inside, you can see the cavity, right? Yeah, cavity is basically that is where the fluid dynamics takes place. So you have this inlet, the jet comes inside this inlet, [00:08:00] the jet travels over here.
And because there are some this diverging walls over here. Yeah, it sticks to one of the wall. Then, because there are backflow limbs, you can see, backflow limbs, so jet sticks to one of the wall, the fluid is transferred from this backflow limb, back again, it switches the jet to this direction. Again, it goes to the another black volume and then the jet is shifted to another direction.
So this is how the, uh, how it works. But, um, just for, um, uh, you know, your understanding clearly, I would like to show you a video. This is the geometry of fluidic oscillator. So you can see that there is a inlet, uh, and you know, that red color thing, basically this is a velocity control plot that we generated by doing CFD simulations.
So if I play the video, you’ll come to know that jet enters the, this thing, uh, cavity. You have this oscillations. Okay. Now what happened is that I’ll just stop it over here. So what happens is now the jet is now attached to the bottom wall. What happens [00:09:00] because of it, because it is attached to the bottom wall, there is a mass flow rate takes place inside the bottom backflow limb.
And this Mass actually tries to switch the jet to the other direction. So, okay. And this is how it oscillates. Yeah, this is continuously oscillates. Okay. What is the Like the real time and this what happens. I mean, now it’s the simulation that’s like a few seconds, but is this also how it would happen in reality or is it, uh, this is exactly what happens in reality.
Okay. So it’s not, uh, it’s kind of a slow. This, uh, is a slow mo video, uh, so essentially, you know, the property of fluidic oscillator is that the oscillation, jet oscillation frequency is directly proportional to the inlet flow rate. So the more flow rate you, um, you know, put inside the oscillator, the oscillation frequency goes up.
What frequencies are we talking about? Just a rough number. Yeah. So we are talking about frequencies from approximately one Hertz to 20 Hertz, depending upon the flow rate. So you can for the device that I’m working on has a oscillation [00:10:00] frequency, roughly for one LPM, one liter per minute flow rate. It goes one Hertz frequency.
Okay. Thanks. So, um, you know, these simulations, so I, I, I was going to, this is fluidic oscillator one device that I’m working on, and there is a second interesting device that is called as vortex diode. So vortex diode is another, um, fluid dynamic, another fluidic device that has a different phenomenon to it.
Now, what is a vortex diode? The name suggests it has, it is, it has a vortex chamber and there is, I can show you the device again. So this is the device, very small. So it has, uh, It has an actual outlet. Mm-Hmm. , right. So what happens is that when from the inlet, the flows come inside. Comes inside, and because of this, um, this profile, you know, yeah.
Uh, the flow, uh, the highly, uh, RI flow gets generated in this chamber. Because it is coming from tangent inlet of the circular section, section, right? So the flow, uh, the flow gets set up, uh, uh, circular flow gets set up inside the vortex chamber. Now what happens is that once the circular flow, the sw swelling flow is developed inside the vortex chamber.
At the core of the vortex, the [00:11:00] pressure drops below a pressure. Okay? Yeah. Now, once the pressure drops below, upper pressure, uh, cavitation takes place. So cavitation essentially is phase transfer phenomena that happens, you know, at at a certain pressure. So we use working fluid as water. So water has dissolved air into it.
So what happens is if you reduce the pressure, first the degassing of air takes place because water super saturates. Right. And for the reduction of the pressure, what happens is that water tries to vaporize because you are reducing the pressure, vapor pressure. Yeah. And it generates bubbles and these bubbles, uh, they are continuously generated and they burst downstream.
And because of this bursting, we have shockwaves generating over there. Yeah. And the shockwaves we are harnessing for multiple things, uh, for processing applications. Okay. So you’re basically, you, you generate this, this. And then in the center, you generate bubbles, basically. Okay. And then your bubbles, they burst.
Yes. And through this shock waves that are generated by this, you can apply for several things. Yes. That’s how it is. So [00:12:00] these are two devices. So what I do, how do you, how do I study these devices? So first I, I do is that I do, uh, use computational fluid dynamics approach to study flow in these devices.
Okay. So you do computer simulations. Yes. Computer simulations. Did you simplify them? Yeah. Awesome. Awesome. In short, yeah. Computer simulations. Now we use the one of the most beautiful equations that has ever been. It’s a million dollar problem still at Clay Mathematical Institute. So if you solve that equation, you get a million dollars.
Okay. Right. So that’s Navier Stokes equation. And, um, you know, this Navier Stokes is one of the beautiful equations. So actually, you know, you have a geometry, you apply boundary conditions and you get, get the flow inside a device. So before even doing an experiment, we know how the flow is going to take place.
So we are actually in a certain sense, time traveling in fluidic devices. Okay. So this is getting a bit like interesting here. So we have a magic processes and time traveling science podcast. And yeah, I mean, so you actually predict flow. So essentially, uh, you know, uh, Navier Stokes has that capacity in a certain sense.
We are seeing future. We are time traveling in devices. We are time traveling in flows basically. So suppose [00:13:00] there is a geometry and if you, if you apply, you know, give some flow rate to this device, what would happen, right? Of course, these, uh, these Navier Stokes equation are very simple in terms that the basic law behind is just mass conservation and Newton’s second law.
But because of this fundamental, uh, theory of mass conservation and Newton’s second law, we apply it to fluid flow and we get something called, uh, uh, equation beautiful, as beautiful as Navier Stokes, uh, which does not have an exact solution till date. And it was originally, it was originated maybe in something, uh, something in 1800s.
And we don’t have an exact solution. And if you solve, solve and get an exact solution, you get a million dollars. The price is still open this year. Go like, no, no. Um, that’s how, uh, the, um, Navier Stokes. So basically we solve Navier Stokes equation, uh, to Uh, get, you know, predict flows in these devices. And then what we do is that as we are simulating flow in these devices, we simulate along with flow.
We, of course, we simulate mixing, we simulate residence time distribution, which is essential for process engineering applications. So we use [00:14:00] powerful computer simulations. Then we optimize the geometry so that we get a certain degree of mixing or residence time distribution or what the process, how to satisfy the process requirement.
We do these kinds of simulations. We optimize the geometry then we have a geometry. Now suppose for example, there is a geometry, uh, which has a mixing intensity 70%. And we want to raise it to a hundred percent. So there should be some geometrical modification modifications that we need to do. And due to this geometrical modifications, you can increase the mixing intensity.
So instead of constructing and manufacturing the device, we do it through computer simulations. And once we have an optimized geometry, we manufacture it and we test whether it is correct or not. Okay. So in your group, you’re both working on the computational side and on the actual. Experimental side.
Let’s say experimental side. Okay. You already showed a few fluidic devices and you talked about it’s important for a lot of processes. Can you give me like [00:15:00] two brief examples of processes, like, you know, why, why should we care about fluidic devices? What are interesting processes? What are, I don’t know, important industrial applications?
Just So, um, we, I’m also working on the application of the devices. So my application is concentrated on, uh, crystallization, anti solvent crystallization. What we do is that we do have model crystallization systems that are already in place. So suppose we have, uh, we have to develop any new reactor. Uh, we already have model crystallization system that we use to test these devices.
So we, what I’m working on is anti solvent crystallization of paracetamol. So, okay. And this is about how to produce. paracetamol or how to make paracetamol more, I don’t know, efficient in its effects in the body or Like what I am focusing is on particle size distribution of paracetamol. So what happens is that, you know, uh, anti solvent.
So what is anti solvent crystallization? So we have a saturated solution of paracetamol in, let’s say methanol and water mixture. So the solution is saturated now. Now you add anti solvent into it [00:16:00] and disallowed is in this case of water. So you have water and methanol mixture. You saturate that solution with paracetamol and the paracetamol is completely dissolved.
Now, now you add water as an anti solvent again. Yeah. So what, as anol, what it does is basically it reduces the solubility of paracetamal inside that solution. Yeah. And now Paracetamal precipitate out. It crystallizes out. Sorry. Yeah. Okay. So you get paracetamal para powder essential, right? The crystals in the, in the crystal.
Mm-Hmm. . Yeah. So, uh, now the crystal size distribution of the forming paracetamal crystals depends upon how, uh, depend upon the intensity of mixing. Right. So, uh, in, in the, in recent study, uh, we were able to show that, you know, uh, we compared our performance of fluidic oscillator with a stirred tank reactor and we had significant reduction in particle size because what happens is that the, the more efficiently our anti solvent and solvents are mixed.
The more, um, mixed it is, the more, uh, nucleis will form of, uh, paracetamal. Uh, because it is completely mixed. I mean, we can assume that it is completely, it is definitely not completely mixed because there is a distribution. Yeah. If it’s hundred percent mixed, it’ll be just a mono dispo, uh, particle size.
But, uh, you know, uh, so in what we did was we compared stir tank [00:17:00] performance with fluid decos later and we had significant reduction in, in the particle size and even, uh, spread of the particle size was also reduced. So we use fluid decos later for that. Okay, and this reduction in particle size is good because it results in better Packing.
Performance. Packing? Yeah. Packing. And even because, you know, particle size, uh, so packing, yeah, basically essentially it is packing. Packing of the, you know, tablets and all the stuff. So particle size is essentially what pharmaceutical industries are looking for. So that is one thing. Okay. And now, uh, this is about fluidic oscillator, right?
Because it acts as a good mixer. Why it is acting as a good mixer is, I, I, I’ll draw, I’ll just pull out another video how mixing takes place in, in fluidic oscillator. Okay. All right. So now the same oscillator, right? So we have, uh, I am, I’m, I’m, I’m not sure if you are able to look, but, um, if you see the inlet, there are two segregated steam, one is blue color and one is red color at the inlet, right?
So we have two segregated streams of, let’s say one is tracer and one is water. So we are [00:18:00] injecting this tracer and water inside the fluidic oscillator. Okay. So the red is the. Make sure and the blue is water or whatever in the, in the sense in simulation purposes, this is just plain water water. So how do you see water and water is mixing with water.
Numerically that water is different. It’s tracer, but it is numerically water. So yeah, I mean you can see this animation how, how this, um, you know, this happens. So I can start this. So see, now you can see that, uh, the oscillator is green, which essentially means that it is 50 percent water and 50 percent tracer, which essentially means it is mixed.
Okay. So you start with, you know, the blue on top, the red on bottom. Um, so they’re completely separate. And then. Once you’re in the device, everything is green. So everything is completely mixed because, you know, there is a vortex that generates and there is a warming of vortex and shedding of vortex. And because of this persistence forming and shedding of the vortex mixing takes place.
So this is fluidic oscillator. So we use fluidic oscillator as a good mixing device in anti solid crystallization to have better control over the [00:19:00] particle size distribution. Uh, we were able to prove that after a certain, uh, flow rate or the mixing intensity of fluidic oscillator reaches a saturation of a hundred percent.
Okay, so you can actually reach hundred, you know, and a lot of processes often you try to reach hundred percent, but maybe it’s not possible, but okay, cool. This sounds all very, very cool and interesting. And I guess there are a lot of super interesting applications and I hope you will show more of your different devices and simulations once you take over the Twitter account.
I’m interested now. So how did you get there? How did you become a PhD student working with these fluidistic devices? at the University of Limerick? Well, it’s a very long story, but, um, you know, I started my, I did my bachelor’s from, uh, as, as I’m, I’m a mechanical engineer by training. I’m a bachelor’s in mechanical engineering.
Later on, I was working in manufacturing industry for a year. I was working in foundry, uh, and machine [00:20:00] shop. Uh, then after that, I changed that job to teaching academia. You know, I was teaching subject of mechanical engineering. After that, you know, I switched that and I opted for master’s. And it is in masters that I actually found this very interesting, um, you know, I found a project to be done in one of the research laboratories in India, which is a government laboratory.
It is National Chemical Laboratory in Pune. So I was working with Dr. Amol Kulkarni. He is one of the pioneering scientists in, you know, chemical engineering and reactor design. So over there, I was doing my master’s dissertation where I worked in micro reactors, micro channels basically to, well, you know, to, um, unify flow flow unification of uniform flow.
I was working in unifying the flows in parallel micro channels so that, uh, you know, uh, that dissertation basically actually. It actually grew my interest to pursue my research in fluidic devices. So over there also I was doing simulations and experimental validations of these devices. And then after my masters, I continued my research over there in CSIR, National Chemical Laboratory, Pune, where I worked with static mixers.
[00:21:00] impinging jet reactors, impinging jet reactors for precipitation, anti solid precipitation, then static mixes for different applications, and even flow distribution. I was designing manifolds for unifying flow distribution in parallel channels. So after that, you know, I was, I was, I was very much interested and clear to do my research in fluidic devices.
When I was searching for positions, in the meantime, I joined industry as a CFD consultant at tridiagonal solutions Pune, where I was doing Just simulations, nothing else. I was doing industrial projects like tanks, lands, and everything. I mean, I was doing everything that could be simulated. I was simulating those things.
And that was my profile. So after that, you know, then I got an opportunity to join my PhD at Bernal Institute at University of Limerick. My project is funded by synthesis and solid state pharmaceutical center that is SSPC. It is a science foundation Ireland research center for pharmaceuticals. So I’m doing my PhD under Professor Vivek Ranade.
He’s another one of the pioneering names in in computational fluid dynamics and chemical engineering. [00:22:00] So I work in multiphase reactors and intensification group. So our entire group actually, uh, works, they work in multiphase reactors. And of course, intensification, we try to intensify these processes by introducing different periodic devices.
So that’s what we do. And that’s the journey so far. Okay, cool. So you So you worked basically in a similar field from your master’s on, you already have some industry experience. So not just, not just academic experience, how’s that change? Like how’s that to go, you know, from university into industry, back to university?
Is it, is it very different, you know, working cultures or is it? That is different. It is definitely different. I mean, in university you have, uh, uh, you know, the working style and in the universities and academies are different. So you actually have very new things to do well in industry. Sometimes I, I personally, my, my personal experience is that industrial job gets a little bit monotonous.
I am not saying that this is wrong, but yeah, sometimes it actually happens. Yeah. It’s a little bit monotonous, but, uh, I think research is more lively. So, yes, that’s [00:23:00] how it is. Then I hope that you, you know, can continue to have such an interesting, interesting job. How long have you been doing your PhD now?
I’m in finally, and I’m about to submit now. Oh, okay. I’m not going to ask any more questions, you know, when you’re finished, nothing about your writing. We are not going to talk about this, it’s okay. But good luck. Thank you. Okay. So we already had a lot, but different, you know, fluidic devices, we already saw a few and you’re already, you know, what I learned has worked in different places, different countries, even on, on this.
So on this podcast, we have a section which we call in other words, and for this, I would like you to pick just one research project and explain it to us in simple words, please. Thank you. Yeah, so the whole idea of using and optimizing fluidic devices to cater to specific process requirement is a little bit intriguing.
I mean, I was I was a little bit interesting that so. So what [00:24:00] we do is that what we did basically and what I actually, uh, you know, we recently published a paper in chemical engineering journal last year and we were able to show that, um, you know, using this devices to augment stirred tank reactor, standard stirred tank reactor to have direct control of particle size distribution of forming crystals.
Um, that is one of the, uh, fluidic devices. So that is one of the reasons that I am, I know very much, uh, I was, I’m very much proud to say, I mean, I would use the word proud that, you know, this is, this was the first time that anybody had augmented stirred tank reactor. So what we did basically was, um, you know, uh, we use the same model system of anti solvent crystallization, methanol, paracetamol.
And initially what we did was we used a stirred tank reactor, a standard stirred tank reactor, uh, which, which hosted a saturated solution of methanol, paracetamol, and we added water as an anti solvent. So then, uh, after the process was completed, we, uh, measured the particle size distribution. Then what we did was, you know, uh, we tried to, uh, we, we had a saturated solution inside the stirred tank reactor and we pumped it [00:25:00] out from the stirred tank reactor and put it back inside the stirred tank reactor.
So this was a closed loop. So you, you took, you, you pump out the solution from the stirred tank reactor, you pump it in again. And now here, what we did was we added anti soluble inside that loop outside the stirred tank reactor. Now we had a significant reduction in, in particle size distribution. Okay, just because we are adding the anti solvent outside the stirred tank reactor inside a t junction mixing little bit enhances and because of this enhancement enhancing enhancement in mixing you have reduction in particle size distribution and the spread of the particle size distribution goes down so now over here we use a simple t junction now instead of t junction we used oscillator and diode the oscillator we used so with oscillator the particle size came down and with vortex diode it came down again Now, one interesting thing that I forgot to mention is that, uh, you know, that the vortex that can create shockwaves because of cavitation, the mobile, because of the collapse.
[00:26:00] Now, um, you know, most of the pharma industries, they use to control the particle size distribution. Uh, what they do is that they use a wet meal or a dry meal. A milling machine, basically just a mill, right? The, what they do is that they inject a solution of, uh, let’s say with particles inside and a solution they inject into the wet mill and then they mill that, uh, crystals.
They mill it literally. They break those crystals. Okay. Yeah. Use the particle size. So now one interesting application of vortex diode is because it generates shockwave. It’s actually, uh, these shockwaves can break crystals. They literally break crystals. So instead of the physical milling, you can use the shockwaves formed in your liquids.
So we use that. So, uh, because we used a vortex diode in that, uh, that study, there was significant reduction. I mean, it was visible, uh, visible reduction in particle size distribution so that we were able to publish in chemical engineering journal recently. And yeah, I mean, that is one of the research that, uh, that actually cemented that, you know, these fluidic devices work.
Okay, I think you’re very right to [00:27:00] be proud of this, just because I think we haven’t talked about this now. Um, can you just give me a brief, like, order of magnitude if we talk about particle sizes? or particle size distributions, but what is the size roughly we’re talking about? Is it, you know? So, uh, this, uh, I mean, in this, uh, this CJ paper, basically, you know, uh, with Stirltang detector, we had particle size, our average mean size of particles basically was something around 150, 140 microns.
And then with just simple addition of T junction, uh, that particle size reduced by 20 microns. With fluidic, uh, interaction of fluidic, uh, oscillator, it reduced further by 20 microns. And then with our vertex, it’s further reduced with 20 microns. So we reduced from particle size from 150 microns to let’s say 100 microns, which is not significantly more.
Uh, I mean, it’s not significant reduction. Uh, but, uh, one thing is that Uh, use one more advantage of using fluidic devices that you have this process to be repeatable. I mean, uh, I have, I, I, I actually published this with error bars, uh, in, in, uh, error bars over the particle size distribution with these devices.
So what happens is that, uh, there was, there is this, this research has been published long back in nineties, I think nineties or eighties that. Where you add your anti solvent or where you add [00:28:00] your second component inside the stirred tank reactor actually, actually has different mixing. So if you add it near impeller, you add it over the surface of the water or you add somewhere inside the stirred tank reactor, it changes.
You cannot have a repeatable process in stirred tank reactor. It’s highly, it’s, it’s not that I’m not saying that it is not highly repeatable, but it is not repeatable. It is not highly repeatable. So there are a lot of error bars and the spread of the particle sizes should be reduced too long. Okay, yeah, but you say your fluidic devices because fluid mechanics never changes because it’s a virtue of geometry and the geometry never changes.
Yeah. So you have this I mean, if you repeat the process, the error bars are too small. And even because the mixing is good, there is the spread of the distribution also reduces. Okay, so it’s not just You know, mean value reduction, but also the, the spread and so the spread, yes, and reputability. Okay. Very cool.
I think we all figured by now that you’re really like fluid with devices and the research you’re doing on them. And it sounds really cool. And, but there are other aspects of being a scientist and just like, you know, doing your simulations and doing the experiments. So what other aspects do you like about?
Being a scientist, being a PhD student, certainly being [00:29:00] a scientist, uh, I would say it’s incredibly fulfilling. It is incredibly fulfilling for several reasons. Now, first and foremost, is that this endless curiosity. You have this endless curiosity of finding out new things and, you know, and this endless curiosity is somehow is acting as a driving force to carry forward research.
And of course, the pursuit of knowledge. So, you know, uh, every day there is a new phenomena that takes place. And as you know, you know, uh, it’s, Oh, after two, after 200 years, there is no solution for exact solution. So you always have this, um, you know, I’m not saying that I’m going to, but I’m going Because that’s not, that’s not my area, but still, you know, there are a lot, many, uh, beautiful concepts and aspects of, uh, being a scientist that you always find new, um, uh, phenomena.
You always find new concepts which are, uh, very much interesting and it acts as a driving force. And then every day there is a chance to explore something new. So when I was working previously in fluidic devices, I never knew that there is a there was an effect called as quanta effect and the jet sticks.
So I’m using that for a device and using it for processing application was definitely something new that I tried [00:30:00] to explore. Right? And then, of course, there is challenging. The existing idea is one of the aspects of being a scientist. So. I, I’m not saying that ster tank reactor is a bad reactor, but, um, I would say that, you know, using, uh, fluid devices with non-moving parts actually has better advantage of ster reactors.
Mm-hmm. in certain cases. Surely, uh, if not all . And then, um, you know, in a, uh, one thing that personally I feel being a scientist actually overhauls your, uh, um, you know, look, uh. Overall perspective perspective towards life. So the ability of how you pursue your things in real life is slightly enhanced due to just it just as an occupational benefit, if not hazard, I would say.
So, yeah, that is, that is the one of the things, you know, being a researcher. Additionally, you know, unraveling mysteries, conducting experiments, finding solutions to real life problems, is brings to a certain sense, it brings a sense of, let’s say, accomplishment in one’s life and also, you know, on personal life front, definitely science actually makes a good impact, um, on personal life too and, uh, subsequently on a larger spectra, um, you know, to, to, to people and planet.
Right. [00:31:00] So, That’s how it is. I mean, uh, so whether it is through enhancing perception, developing new technologies, including healthcare or addressing environmental challenges, you know, these are all the aspects that, you know, not, I am not working on everything or everything of this, but we as a whole, as a scientific community, we are contributing a certain way for the betterment of society.
And that that’s, I think is the best part of being a scientist. That is very true. And I don’t think I can, you know, add anything to this. You said this very nicely. So I think I would just. Yeah, I should definitely leave it like this. No, but it’s, I think it’s, it’s great. And I, I, I especially also the, the point where you said resonated with me that you, you know, continue learning and, you know, there’s always, there’s so much still to discover.
It’s not like we, you know, figured it all out. That’s definitely not true. And it’s. Yeah, it’s very, very cool to say, stay curious and play. And yeah, hashtag stay curious. Okay. Um, yes. So this sounds all very good and like, you’re really, you [00:32:00] know, enjoy being a scientist and enjoy your research, but you know, if nothing’s perfect, so if you had three wishes to improve your research.
experience, what would they be? One focus thing that I have experienced, you know, I’ve been in doing my research since like, let’s say 2015, it’s been over like eight years now. One thing that I definitely want this to, and I think this is true across every, every field, bridging mechanism between interdisciplinary fields.
There has to be a bridge so that, you know, you know, there has to be certain mechanism that we are bridging interdisciplinary fields. So for example, if I talk about fluid mechanics to a chemist, He’ll not understand anything. So, you know, uh, there are, there are certain things I’m not saying that everybody doesn’t know that, but, uh, you know, if your reaction, let’s assume that, you know, if your reaction time is this very less, very less, and your reaction, your reactor’s mixing time is more than that.
It is not going to, I mean, that reactor has [00:33:00] no, uh, that, that, that reactor has no role to play over there. So that’s, that’s, that’s You know, also to, uh, bridging these gaps of interdisciplinary fields. Maybe, you know, we, we might to some extent, uh, uh, enhance the impact of our research. Mm-Hmm. . So bridging is essential.
So if there somebody is developing a process, uh, we will, we try to study that process and then we try to make it continuous. But people are still stuck in, in, let’s say, the conventional process and they don’t wanna change. Okay. Change. So chemists who are listening to this, please go back to the beginning of the podcast.
No, I can’t, I don’t expect, no, joke aside. Um, I agree very much. Like I think there, you know, you speak your own language and every sub field and you have your own, you know, bunch of literature and sometimes, you know, even among fields that So, yeah. Bye. In principle, neighboring, not always so easy to, to talk to each other and to get the others [00:34:00] true to rate the relevant literature and so on.
So one example that I would like to give is that, you know, in mechanical engineering, there is a, there is a device called a heat exchanger, so normal tube shell and tube type heat exchanger. Now, uh, if you, we can use that shell and tube type heat exchanger as a reactor too, but Uh, the velocities and all the tubes are constant.
We maintained, uh, mechanical engineers know the calculations to maintain the velocities in the, in the tubes constant. But when it comes to application for this chemical engineering, you want residence time distribution to be constant, I mean, all inside all the tubes. So this, if, if we combine these two, these things, we can use a shell and exchanger as a reactor too.
So that is one example that I would like to go to interdisciplinary fields. Okay, that was your first wish. What’s your second one? Second is, uh, this is a dream come true. Basically, I work in simulations. Give me a supercomputer. Okay, I’m sorry. I’m sorry. I should have said this in the beginning, but we actually Actually [00:35:00] cannot make your wishes come true.
We just ask . I cannot give you a super computer base. Sorry. No, that’s fine. So basically know unlimited access to cutting edge technology. So of course the un restricted use to, um, uh, experimental tools and, uh, computational tools is what, uh, uh, sometimes it is restricting, uh, because, you know. The amount of, um, you know, why am I saying this, uh, of this computers things is because sometimes you know what happens is that there is one cluster where everybody is using and sometimes the job goes, it goes in queue and there’s a lot of time delays here and there.
So I think unrestricted use for, um, for computational and experimental tools is second wish. Definitely. I would like to have that access to all the tools and yeah. Very understandable. And your third wish? I’m sure you’re going to agree with the third wish. Sustainable research funding and recognition.
Yeah, it’s, I think Like we’ve asked this question a lot of [00:36:00] people and a lot of people say they want more money, like, not for themselves, but for their research. Last but not least, of course, money matters. So yes, money. And then I recognition is another thing that actually I write to impresses and I love the book that science talk is doing in this field.
And I truly appreciate you guys, because that is not something that you know, people do to recognize, give recognition to. At least researchers. Unsung heroes, basically. We try our best. Thank you. Okay, so your three researches were, um, more interdisciplinary or better interdisciplinary work, um, supercomputer and more stable research funding.
Basically, all three very valid. I really hope they come true for you. Or, um, I mean, the first one maybe comes through. for all of us or for the whole research [00:37:00] community. I think that’s a very good point that becomes more and more important and I hope we, you know, managed to get there. Okay. Um, thank you so much.
Um, apart from this podcast, you will also have One week access to the real scientist’s nano Twitter account. I’m still saying Twitter. That’s what I mean. Right. Um, so doing this week, what can the followers expect? I’m planning to do whether to this curation week. I mean, when I will be the handling the Twitter account or the ex account.
Uh, so, uh, first I’ll, I know we’ll introduce a group of multiphase reactors and intensification, our philosophy and what are the areas which we are working in, and of course, then I’ll write all the devices that we are working on. And then of course, there will be some interesting applications that will be disclosed.
So how can you use these devices for, um, for different applications. So we are working on, let’s say, um, we are working on milk production, milk, uh, waste waterization of [00:38:00] the dairy industries. We are working on personalized emulsions and then we are working on crystallization too. And so these are broad spectrum of applications that we do in our group.
And yes, so what we do is that, you know, I will try to first introduce the group and then eventually, uh, disclose few interesting things that we are doing as a group. And also if, um, uh, I’m going to I’m not sure, but I will also write something, something interesting in, in, in fluid mechanics. Uh, that is my plan for, for the week.
Sounds very good. And I think what I figured now is that these processes are important for so many different applications and processes that I didn’t even know, you know, you would use fluidic devices for. So very much looking forward to this. Thank you so much for speaking with me. And looking forward to your week on the Twitter account as well.Yes. Thank you very much. It’s, it’s a pleasure. I cannot thank you more. Thank you very much for this opportunity again. Thank you. And thanks all for listening or watching. [00:39:00] Goodbye.
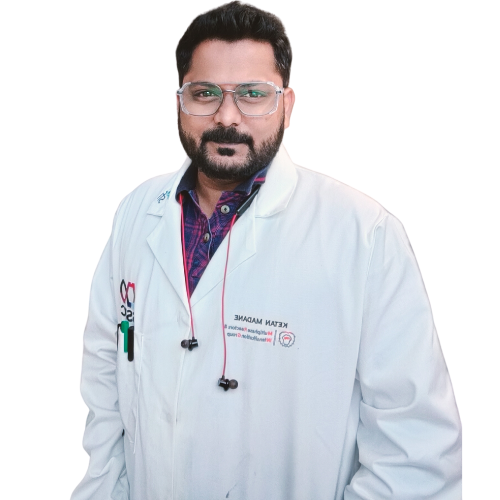
The Magic of Flows
Ketan is a PhD researcher at the University of Limerick (Ireland).