What to Expect:
In this episode, Dimos Katsis shares his journey from joining a solar car team in college to becoming the director of power electronics at Power America. Dimos provides a deep dive into wide band gap semiconductor technology and its transformative impact on electric vehicles, aerospace, and beyond.
About the Guest:
Dimos Katsis
Dimos Katsis is the director of power electronics at Power America. With decades of experience in power electronics, he has been instrumental in advancing wide band gap semiconductor technology, which plays a crucial role in electric vehicles, aerospace, and other high-tech industries. His work involves collaborating with industry leaders and researchers to drive innovation in power electronics.
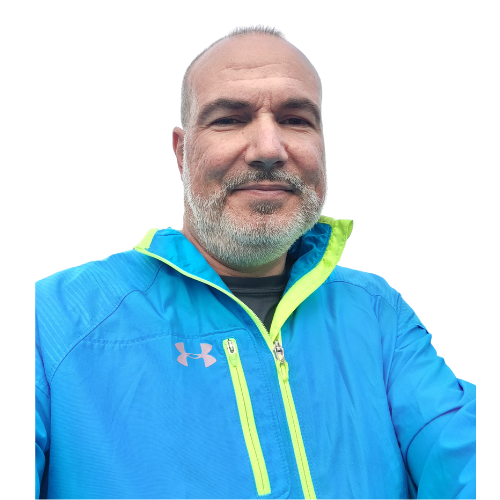
🌟 Key Takeaways from This Episode:
- Wide Band Gap Semiconductors: Revolutionizing power electronics for electric vehicles and aerospace applications.
- Career Journey: From early involvement in solar car teams to leading research at Power America.
- Future of Power Electronics: The potential of silicon carbide and gallium nitride in transforming various industries.
🔬 In This Episode, We Cover:
Dimos’s Research :
Dimos’s work focuses on advancing wide band gap semiconductor technology, particularly silicon carbide and gallium nitride, for applications in electric vehicles and aerospace. These materials offer superior performance compared to traditional silicon, enabling more efficient and reliable power electronics.
Dimos’s Career Journey :
Dimos’s career began with his involvement in a solar car team during college, where he worked with NASA-developed solar energy harvesters. This experience sparked his interest in power electronics and led him to pursue a career in this field. Over the years, he has worked with various organizations, including General Electric and Blue Origin, before joining Power America.
Consortiums and Collaboration:
Dimos discusses the importance of consortiums like Power America, which bring together industry leaders and researchers to share knowledge and advance technology. These collaborations are essential for driving innovation and ensuring the development of cutting-edge power electronics.
Life as a Scientist- Beyond the Lab :
Dimos values the collaborative nature of scientific research and enjoys engaging with the global scientific community. He is passionate about teaching and mentoring the next generation of scientists.
Dimos’s 3 Wishes
- Increased funding for basic research: Dimos emphasizes the need for more financial support to advance fundamental research that can lead to groundbreaking discoveries.
- Forward-thinking investments: He advocates for investments in technologies and infrastructure that can benefit future generations.
- Bridging the gap between scientists and the public: Dimos believes in the importance of improving the relationship between the scientific community and the general public to foster better understanding and support for scientific advancements.
Dimos’s Time on @RealSci_Nano :
Dimos will be taking over the RealSci_Nano Twitter account to share his research on wide band gap semiconductors and their applications. Followers can expect to learn about the innovative techniques and materials his work focuses on, as well as insights into the future of power electronics.
Join the Conversation
Follow & listen to “Under the Microscope” on Spotify, Apple Podcasts, Castbox, Amazon Music, Goodpods.
Got your own favourite podcast app? Follow the RSS link to find Under the Microscope.
Watch the video of all Under the Microscope episodes on The Science Talk’s YouTube channel. While you are at it, make sure to subscribe to our YouTube Channel.
Learn more about Under the Microscope – https://thesciencetalk.com/under-the-microscope/
Love what we do? Support us:
Be our Patron – https://www.patreon.com/TheScienceTalk
We also offer partnerships to scientists, research institutions, industry, funding bodies & societies.
Get in touch to start the conversation.
Transcript
[00:00:00] Hi, everyone. My name is Pranavati. Welcome to yet another amazing episode of Under the Microscope. Today we have with us Deimos Katsis, who is a director of power electronics at Power America, and he will be able to explain to us what all of that power means. So before that, welcome Deimos. It’s a real pleasure.
Thank you, Pranavati, for inviting me. I’m always happy to talk about my story and Uh, the research that’s going on right now to help with, um, making electric vehicles and, uh, technologies around those, uh, much better than they’ve ever been before. We’re in the middle of a huge, I guess, a huge change in electronics that, that many people don’t realize is happening.
And that’s, uh, that’s an exciting time to be in right now. So we’ll talk about that. Speaking of electronics and the use change that is going on at the moment. So explain to us, like, what exactly, where do you come in? Where does Demos or where does Power America come in, in the electrical [00:01:00] vehicles or electrical mobility, so to say, what kind of research, like from the research point of view, where do you come in?
Of 89 industry members, mainly from Europe and the US, who have agreed to share information technologies and research in the advancement of wide band gap semiconductor technology. So, I know that’s a mouthful. And what we can do is we can take that apart. The consortium is companies that are willing to give their time and energy, and then to receive also funding to do research, to advance all types of technologies, not, we’re not, we’re not just talking about electric vehicles, but the technologies around there.
So electric vehicle charging, which involves power supplies. The same type of power supplies you see in data centers. So we have companies that support that near and dear to your heart. We have material science companies that make the packaging materials and the components that hold the electronics together, which is [00:02:00] almost as important as the electronics themselves, I would say, and sometimes more important because we need the enabling technologies to get it.
These, the, the silicon carbide and the gallium nitride into electric vehicles as one application, uh, aerospace is another big consumer of the same technologies and because now we’re seeing hybrid electric aircraft and aircraft with electric turbines instead of gas turbines on the wings. The same technologies that we see in EVs apply to aircraft, so you could really say the electrification of transportation, and this consortium consolidates the technologies created in these transportation industries through the guise of electric vehicles, but it’s more than that.
Mm hmm. Okay, that’s that’s fascinating. And I’m so happy that this consortium exists so that to to make sure that everyone is aligned and everyone is informed and there is no repeating [00:03:00] of the mistakes and and the work. I think you can learn from each other, the companies, the research groups that can learn from each other.
And did you say more than 80 companies around the U. S. And in Europe and, and in Asia. Yeah. So it’s a worldwide consortia of companies, including, um, companies from Asia, we have, um, companies that manufacture, for example, the world’s largest power supply manufacturer is Delta. That company is based in both Taiwan and in China.
And just like TMA, TMIC, the, um, the Taiwanese, uh, industry manufacturing company of, of, of, of integrated circuits, it’s, which has been the center of the development of extremely high performance. Power power, not just power, but computer chips. So the massive computer chips manufacturers, which are based mainly in Southeast Asia, would be an example of some of the companies.
I don’t think TMTMIC [00:04:00] is in that. In that consortia, but I know the companies that use those components are and Delta is a heavy hitter in that one of the examples of how closed our, our, our supply chains are, how worldwide they are is, uh, Ford makes electric vehicles in Mexico, power supplies made by Delta that come from Taiwan.
So that just is one example of how, where you get so many different bits and pieces. You mentioned that the technology or the batteries basically that are used to run the cars on the streets is similar to the air taxis or helicopters or airplanes or whatsoever. So let’s just go with like a two seater helicopter, right?
This is out of curiosity and you don’t have to answer this question. Of course, let’s say the car, like a standard car, uh, it needs one battery and we can talk about the dimensions more in detail, but if it’s on one battery. [00:05:00] What, what is the number of what scale of batteries are needed for running a two seater helicopter?
Like, is it like two times the battery? Is it like five times, 10 times just to get, you know, the feeling for it? That’s a good, that’s a good question. And I’ll, I’ll give you a A somewhat educated answer because I don’t know what the energy balance is for aircraft. Exactly. I do know that if you’re flying a small aircraft and not this is not a helicopter, but I know if you’re flying like a, a Piper cub or something where it’s a two seater personal aircraft that a private pilot could fly.
I think the fuel economy runs about 10 miles a gallon. It could even be lower than that. So let’s say 1 4th of the fuel economy of a normal gasoline powered small car in Europe, for example. [00:06:00] So then you would need and because that energy density is. The energy density of the battery is not going to be very high.
You’re going to need a lot more battery for that aircraft. Now, because of that, that means the aircraft just got heavier. So we are not going to outperform a normal 2 seater aircraft. We’re not going to make it more efficient. But if we use a rough 4 to 1 math. Then if you have an electric vehicle battery pack that weighs a thousand pounds on a normal car that that then say you need 4, 000 pounds of battery for the for the aircraft to do this and it’s only going to get half the range of a normal aircraft So so that’s those are the kind of numbers we’re dealing with right now, which doesn’t sound very good Wow, maybe I don’t want an electric vehicle.
However, for, for short stops and for quick access, maybe you want to go 10 miles. Maybe you want to avoid traffic in a large metropolitan area. 10 miles is perfect. Then that is an ideal setup because now you don’t need the infrastructure to refuel that, you just need a power outlet. You can also launch that [00:07:00] two seater helicopter from the top of a building.
You’re not going to need to bring fuel and other support equipment. That electric, that electric system is also infinitely controllable compared to a gasoline or a diesel. Or jet fuel powered system or kerosene powered system, which means you’ll have more adaptability to changing air flow and wind patterns and aerodynamics.
So. The, the strength and the enabling technologies that electric propulsion provides sometimes outweigh the inefficiencies of carrying a heavy battery, or, or the, or the other option is, I can’t carry heavy battery so I don’t have much range, but then I can enable short hop operations. However, there are companies including In here in the D.
C., Washington, D. C. area, where I’m located right now, where hybrid electric aircraft are being built. And so the fuel is used to run a turbine, but the takeoff is done totally electric. And then the fuel is simply there to keep that aircraft flying for long distances in the air. And then the high power that’s needed to get the aircraft off the ground is not put on You don’t have to size the engine.
To take the aircraft off, you only need to size the engine to keep the aircraft in flight so that engine becomes much [00:08:00] smaller that that gas turbine becomes tiny instead of become. And in fact, that 1 of the aircraft that I’m supporting right now, because I also consult is. Uh, the engines, the, the turbine is the size of a watermelon, but it’s, yes, the turbine is the size of a watermelon and it provides all the power necessary to run a large four seater prototype aircraft with large set of electric vehicle batteries underneath the belly of the aircraft.
Or it’s, it’s flying, uh, for its entire flight distance. And it is so cool. That is so fascinating that there is also the hybrid version. Of course, that makes sense. It’s, it’s very, I think we are limiting ourselves. Like we are doing this one on one comparison that, okay, my car with, uh, with fuel can go a hundred kilometers in like five hours, a hundred kilometers in 10 minutes.
And your car, uh, an electric car can only go 50 kilometers in 20 minutes. I think the [00:09:00] parameters to define or measure the success and efficiency of an electric mobility option, be it a helicopter, be it a Uh, a taxi, be it your scooter or whatever, the parameters are different. So it’s, we need to change that mindset as well, that it’s not just about getting from A to B as quickly and as fast as possible.
It’s also about the more sustainable option. that you need, the number of stops you need to refuel and so on and so forth. But this is, this is so cool. This is so, so very cool. Oh my God. And I have a bunch of more questions, but let me, let me first understand from you, Dimos, how did, how did this happen?
How did you become the director of power electronics? At Power America, tell me about your career journey. How does one become a director of power electronics at Power America? Well, for me, I, there are not a lot of positions that allow you to, to make a strategic choice [00:10:00] on, on technology. Um, I think it does come with many years in the field.
I’ve been working in, in power electronics since 1991, really, since I graduated from high school. And here’s why. I immediately joined the solar car team and when I started at Virginia Tech in my undergrad and I started working with graduate students who were on NASA programs that were developing the solar energy harvester for the International Space Station, the same electronics that went into the International Space Station were whatever was cast off and didn’t work there.
We took those very gingerly and placed them inside of our solar powered vehicle and used the same electronics because the battery voltage was the same as the International Space Station is a hundred volt battery. And we took those electronics and we were gifted solar From a number of [00:11:00] organizations that had built space grade solar cells.
So we essentially had a rolling platform for testing power electronics for supporting solar powered space station electronics, and in our case, solar powered race vehicle. And so we took that and we ended up building the world’s slowest solar power car. And I know it was designed to make you laugh because indeed we spent so much time building this car.
And when we raced it, it was the slowest of 36 university teams that raced, but we had made a terrible decision. We decided to make the smallest, most aerodynamic car possible. Which meant that it sacrificed the ability to collect solar energy for aerodynamics. One of the problems that electrical and all engineers and scientists in general discover is, you have to make compromises with your time and your energy or your talent.
You have to decide where to put what capability and resource to work. And in our case, The car needed to be [00:12:00] lightweight, it needed to be aerodynamic, because we were engineers that had not had a lot of experience building cars, our car was neither lightweight nor terribly aerodynamic, but we did have those lovely solar cells, the ability to capture energy.
And then we found out that was going to rain, it was, it rains the every day, except the last day of our, of our, of this competition. And this was a competition where we drove across the entire length of the United States. So, on the last day, we had our one sunny day. And on that sunny day, we passed every single car in the race to finish first for that day’s distance.
We were able to, we quadrupled our average speed. And so our average speed being 10 miles an hour became 40 miles an hour, or I suppose 85 kilometers per hour. We were able to, um, essentially drive at normal highway speeds. With this vehicle that was optimized for a sunny day. It also helped that there was a strong [00:13:00] headwind.
Perfect. Our car is aerodynamic. None of the other solar cars were. So not only did we collect, um, some energy, but we were able to manage it better because the weather conditions were favorable, none of the other days were like that, so we still ended up at the end. We still ended up last, but we had one day of true greatness.
And that, that for me was, was all I needed to keep me in, in solar energy. So it’s not answering your question, but it is giving you a little bit of an idea of how we started with, um, going to power America from there. I decided to make that area, my research. So after finishing my master’s degree with the electric vehicle program, I worked at General Electric for a number of years and returned to the same university to work on my, my dissertation.
I wanted to get a PhD. However, I was inspired by something we were doing at GE, and that was Six Sigma quality, quality measurements and all of the technology around making things better. Immediately, when you say that in a university setting, [00:14:00] material science comes up, because It’s one thing to make the technology, but when you make it so that it’s commercially viable, it cannot be commercially viable without it being packaged and packaging cannot occur without material science, because it is the material that is used to bring the semiconductor component that’s silicon, the silicon carbide or the gallium nitride without the materials, you’re not going to realize that and even the development of silicon or gallium nitride is a material science activity.
Thank you. Yes, graphene is very special, but it’s only a tiny subset of the technologies needed to make an electric vehicle. And you would even say that graphene, with all of its usefulness, can be used extremely well in a very narrow area of the electric vehicle, and only then as an option. There are other ways to, for example, do heat removal.
Graphene is an extremely, uh, useful heat removal tra uh, compound. You can oriented graphene has an has the same thermal conductivity, uh, as [00:15:00] as silicon carbide, which or diamond. So you can you can have, uh, amazing heat flows through through graphene. But that’s not what I want to use. In fact, it would be relative to diamonds, since diamond’s also graphite.
So, and, oh, by the way, I was going to say, you can use, you can make transistors out of diamond. That’s another discussion for a little later. Transistors with dry di are you okay? Diamonds and transistors? No, that doesn’t make any sense, but yeah, I understand. I understand what you mean with, um, yeah. Gallium nitride, silicon carbide.
This is also material science and this existed. Decades before the graphene boom sort of came about, um, for sure. So that is, that is the key role that material science is playing and in this, uh, electrical mobility solutions, right? Well, we talked about, um, besides the, the making of power semiconductors.
Through the role of material science, we also talk about this very simple things like encapsulating electronics, making sure that you don’t arc from one voltage level to another, protecting the user from interaction with a high voltage battery pack [00:16:00] requires, uh, just the simple decision of what type of encapsulant, what type of two part polymer do you use that cross links and cures and becomes a protective Barrier between the battery terminals and you if you buy an ionic five from Hyundai or a Kia EV six, you are dealing with an 800 volt battery pack.
So we know that plasma fires start above 230 volts. Can you imagine now 8 or 4 times that voltage? At an 800 volt battery pack and the type of plasma fire that could occur if the two different conductors touch or touch briefly and create an arc because it’s a battery. That arc was never going to be extinguished.
It will be extinguished when the battery completely is is gone. But that’s a one time use battery and the fire is spectacular. [00:17:00] And a lot of people worry about that, and the only way you can avoid that type of fear in the marketplace with electric vehicles is the successful application of material science.
And so something as simple as an encapsulant material that can both contain the battery, conduct the heat while containing the battery, and provide the voltage withstand that is necessary to keep the battery from shorting across its leads, and protecting the battery in the case of an accident. So that a short circuit doesn’t occur, even if an accident occurs.
These technologies exist and are being used in electric vehicles today, and it’s the only reason that we can buy electric vehicles is because of the material science and something as simple as a cross linked polymer that is poured over the battery to provide that next level of protection. And that in its own is an entire science of material sciences is polymer coatings for electronic [00:18:00] systems.
We know that when. Two part epoxies outgas. Sometimes, depending on the composition of that, you can have an outgassing of a corrosive substance. Last thing we want is to corrode the batteries while we’re protecting them. And so, again, these are the type of thoughts that’ll go into an electric vehicle battery.
Manufacturing. We haven’t even talked about making better batteries, which is what you did. We’re just talking about making batteries, a better product or the consumer. So the consumer isn’t scared. So the consumer can say, Oh, Pranoti has made an amazing battery, but I’m scared of this battery now because it’s so amazing, but not anymore because it’s been coated with a lovely polymer and the polymer is protecting me.
Right. So there is materials everywhere, literally everywhere, starting from the core of the battery to, uh, the entire package, like everywhere there is, there are materials and materials. Um, it’s, it’s, this is really, really [00:19:00] fascinating taking like a deeper dive with you demos into the world of batteries and what are things to keep in mind.
So, No arcing, no corrosive, uh, outgassing, no, basically safety of the battery and also of the people who are around that battery and everything that is around that battery and long term stability. So some of my research included the outgassing measurement of polymer compounds used to encapsulate electronics in order to avoid.
The long term aging, what we did, or the effects of long term aging, we characterize the effects of long term aging by putting these power semiconductors with the polymer encapsulants over each, each semiconductor into an oven, raising the temperature far beyond it. what you would see in an electric vehicle environment and then letting them bake.
And then we would take out the electronics and apply extremely high voltages to see where arcing would occur. And then we used other devices to [00:20:00] measure something called Corona. And this is that have you ever run under power lines, maybe on a morning jog, or maybe you’ve in the morning, you’ve gone out into a park or somewhere and you’ve seen some power lines overhead.
Have you ever heard a little like sparking or sparking? Yeah, like static almost coming from the power lines like they’re they’re they’re shivering. Yeah, like yes Yeah, mm hmm. That noise is called Corona and Corona Corona or Corona different Corona. Yes. This is the Corona that doesn’t involve the lime Okay.
Or, or, uh, uh, a shot. Oh, yes. Yes, or that. Exactly. So, so, none of that. This is the corona where charged particles are ionized in the atmosphere and create tiny arcs, tiny, tiny voltage lightning strikes. To ground. [00:21:00] And so you’re hearing tiny lightning strikes when you hear power lines overhead at high voltage that can occur inside electric vehicles without the proper material science applied to the batteries.
Well, we don’t want that. No, we don’t. Because over time, what will happen is those ions will draw the metal out of the bar and create a bridge. We’ll create a metallic bridge that looks like the branch from a tree or a dendrite. You’ve probably heard that, the Greek word for branch from a tree. And that branch, as soon as it touches the other, the other conductor on the, the battery, in your case, if you’re dealing with electrolytes, you know, if dendrites occurring inside the battery.
But I’m talking about dendrites occurring outside the battery. Oh my god. So it’s basically ions finding the way, finding a way. And creating a bridge out of metal. Yeah. Oh my God. That this is everyone is scared of the power of AI. They should worry about the materials, the power of material science [00:22:00] and the ions and everything.
This is, this is really, really, really scary. I mean, it’s fascinating as well. It’s good to know of the good to be aware of what can go wrong so that you can prevent it from happening and tested a bunch of times in the lab and also in the production line. So if you have to pick one research project that you’re most proud of or the most fun or quirky one, could you pick one, one, okay, just one and explain it to us in the section we call in other words, Okay, I will pick the quirkiest research.
And that was a team that I worked with at the army research laboratory. And we published this in an open journal so we can talk about it. It was to take radioactive tritium. And to use it to illuminate tubes of copper zinc sulfide, copper doped zinc sulfide [00:23:00] to make the tubes glow green from the inside out, and then attach the tubes to certain cylindrical solar panels.
to generate tiny tritium powered batteries that would last 20 years. Okay. I need more information about, uh, I have so many questions. Why, why did you, like, how did, how did I get involved with nuclear power? That, and also why does it have to glow and where does the solar come in, in all of this? If you have the nuclear isotope, then why, where does the solar, and also why, why do you need, just, I mean, other than the fun of it and the quirkiness of it, why?
Well, we had a request. To build a sensor that would last 20 years and electronic sensor that could broadcast measure information like [00:24:00] temperature, moisture, the types of information that you could measure in the field. Maybe you could mention even pick take a GPS coordinate, make, make a measurement and then store that in a microprocessor and then maybe once a month store enough energy to create a tiny radio transmission.
Once a month. Okay. Yes. So in order to do that, you’re, you’re probably only going to harvest microwatts, a few microwatts of energy. And that was perfect for our application. There’s not a lot of 20 year energy storage systems. And even after 20 years, you’re still generating electricity. If you think of the Voyager space probe, the one that’s left the solar system, Voyager 2.
It’s running on a plutonium RTD. And so what it has, it has a small charge of plutonium and thermocouples that are, that are, Essentially built [00:25:00] around this plutonium heat source and by keeping one side of the thermocouple cold and the other side warm thermal junction, you are generating a thermal battery, an electrical battery that’s operated from heat.
And that system is what is still powering Voyager to this day and Voyager launched in 1974, I think, or 1973. So Voyager has been in space for a very long time, 50 years, but it’s still running on its batteries on its plutonium battery. So this is not as dangerous as plutonium. Tritium is actually quite harmless.
It’s a hydrogen isotope that emits electrons, um, beta emitter. And that’s all it emits. It’s just a pure beta emitter. And because it’s hydrogen 3, it also dissipates very quickly. So if you had a tritium leak of the small quantities we’re talking about, it would be essentially unnoticeable. Now, you also have to be careful if you inhale tritium.
The emitted energy, the beta particles would interact [00:26:00] and damage DNA in your lungs. And then you could eventually, maybe after 20 years, get lung cancer. So, you know, you want to avoid tritium inhalation, if at all possible.
Yes, good to know. There’s not a lot of tritium around, so it’s quite expensive. But if you can take tiny tubes, tiny glass tubes, and you use that as a source of light, and then manufacture specifically designed solar cells that can convert that particular wavelength of light efficiently into electricity, then you can create something called an indirect converter.
It generates no heat signature. It, it, it’s, it’s undetectable. Radiation wise, because the radiation is putting out is just electrons like a little cloud of electrons. So you can’t detect it. You can’t smell it. You can’t see it. It’s completely self contained and say long term power source. Wow. Can [00:27:00] we use that for our satellites now?
Like, because I think this will be this is so and also it glows, right? It does glow. It does glow. That is also very important. So the optics of it are also very important, uh, in addition to its efficiency for sure. But can we use this or is it already being used for all kinds of different satellites that we have are also this Chandrayaan, uh, that landed on the South pole of moon to collect samples?
Because I guess that is where you definitely need, uh, sensing over like decades. Uh, also the good news with landing on, on the moon is you can have access to powerful solar radiation as a much better source of light than the very weak light that a tritium tube would generate. And so if you’re going underground, let’s say [00:28:00] for example, you want to go to IO or you want to go to a moon of Jupiter where there are oceans that we know of, then you want Something into the water that can continue sampling and collecting data, but not poison the water.
So nuclear is certain types of nuclear are out. Tritium might be an option, but even then, if something breaks that glass, then you will have bubbled some H3 into that water. Now, it’s very possible that water has H3 in it. Tritium is, is not. It’s it’s the result of nuclear reactions. It’s probably very clear that Jupiter itself is full of tritium because it’s a gas giant and hydrogen is part of that and H3 is is basically a version of that.
So we don’t want to, to poison the planets or the moons that we want to collect information from. And so it’s very, very [00:29:00] scary for me to think about sending nuclear reactors to the moon, but there’s a very serious discussion about doing that right now as the power source that would be used for mining the moon, for exploring the moon and for making fuel on the moon.
As we know, the moon has subsurface ice. It would take a nuclear reactor or a very powerful solar farm built on the moon to, to electrolyze the ice and create the hydrogen and oxygen that would support a moon colony and refuel rockets that would have reached the moon in order to send it back to the earth.
But that’s some of the discussion that’s happening right now. But when we send nuclear. Out to other parts of space, we’re doing it with at least if we’re doing it in an exploration idea, we’re doing it with with a concern that we are poisoning what we’re going to explore and as good scientists, we want to leave no trace.
No, [00:30:00] absolutely. And it’s also, it’s not ethical to, uh, to do that. And a second reason being, it can just come and backfire on us that the moon Lords or the Mars Lords or Jupiter Lords, wherever these, uh, little tubes, little tubes end up, they get angry. And then suddenly there are UFOs everywhere. Um, Yeah, that would be, that would be a bad day for us.
That would be another Monday. That would be a really bad Monday, but lots of, uh, movie ideas here as well by just sending, like, uh, I think those script writers need to be listening to this podcast. Yeah, definitely. We can, we can, uh, we can tag them. Uh, when we are, um, publishing this scary, scary sci fi scripts, no, it’s just preparation, right?
It’s not scary. This is science fiction. You know, you can do anything under the name of fiction [00:31:00] ish. Um, so let’s do that, but let’s make sure that this does not backfire. Fire on the, our existing science and the funding for science. I think that is very important as well, which we will speak about in a bit.
So, okay. Wow. Do you have pictures of those glowy tubes? I’m sure you do. You published a paper? Of course you do. I can there, yes. I’ll send you a link to the paper. I’ll just send you the paper and then we can decide from there. It is published in the Society of Radiation and Material Science, uh, Soma.
Mm-Hmm. and uh, the. Uh, the work, we have, uh, pictures, there are pictures of the tritium tubes. They’re actually come from Switzerland. They’re used in very expensive watches to illuminate the dials. Oh, my God. Oh, my God. Every, every. Every five seconds there is like a podcast idea that is coming in my, uh, mind like, Oh, we can talk about that.
Oh, we can talk about that. That would [00:32:00] be so cool. Okay. Okay. Okay. Okay. Okay. So science is cool. Science is awesome. We love research. We love science, but what else? Do you like about your life as a scientist, Timos, tell me that other than research and making these glowy tubes, which we do not want to send to Mars or moon yet.
So being an engineer with a PhD is a little different, I think, than being a scientist, because what I have to do is very extreme literature searches. To understand what the state of the art was to see if we’re improving the state of the art and to see if an idea really is a novel. So, as an engineer with a PhD, it’s a little more, it’s not as exciting as, say, working on something that no one has ever seen before or rediscovering graphene.
I think that’s where great [00:33:00] science comes in. comes up with an idea that no one else has ever thought about. I think that’s true science. I don’t really think I’ve done anything like that. I might have combined a few ideas together, maybe to make something new. And people, patents happen all the time.
People invent new things all the time. And that’s, that’s lovely. And great inventions are in their own way, Um, a form of science, but not research and development of completely new things. So I don’t know, I, I, so I don’t, because of that, I don’t think of myself as much of a scientist as just an engineer plus.
So an engineer plus research guy or an engineer plus someone who spends more time at the library than they should. That’s maybe more my, my application of research, like, for example, um, at, I worked at Blue Origin, which is a space tourism [00:34:00] company owned by Jeff Bezos. And I worked there for six years. And as a principal engineer, my job was to not just say, this design is good, but will this design survive in space?
And so we spent some time at the Lawrence Berkeley National Laboratory in their synchrotron laboratory, spinning heavy ions to the speeds that you would see in space. And beaming those heavy ions like krypton or argon or silver into our electronic packages and seeing if the electronic packages would survive this artificial space environment that we’re generating on the surface of the earth.
And so it’s, this is an interesting material science question because we’re taking a semiconductor. A material that we’ve manufactured on earth by crystal growth and doping and ion implantation and then implanting space ions. To see if what we built will survive the onslaught of this [00:35:00] hailstorm of space weather and the, and the result of that involved doing the research, the literature search, understanding the science behind electronic failures and how they are affected by space weather and then linking space weather with the probability of failure for our designs.
at Blue Origin. And so that was part of our space effects group, which involves more science. And so people immediately say, okay, it’s not just enough to be a rocket scientist, but you now have to know the material science interactions of space weather with the electronics that have been manufactured.
And not only that, but the polymers that are protecting you can be infused with boron now. So you take boron powder, mix it in with your two part polymer, and the boron’s high cross sectional area to nuclear radiation will reflect back the nuclear particles, or the [00:36:00] ions, or, or redirect them, and then give you some amount of protection for your electronics.
So that’s where the engineering comes in. How do I protect myself? Yeah. How do I use materials to protect my stuff, my materials as well? Is it fair to say that you love the part? Of using your knowledge as an engineer or as a scientist to basically bridge the gap to some extent, or to protect what scientists to make sure that it is scalable, it can go on the production line.
It is the technology transfer aspects of it. So that. I mean, I don’t, you know, that you have so much experience that I don’t want to limit your experience just with one title. What else do you like about being an engineer? Let’s go with that. Other than protecting our materials. I think as an engineer, there is a worldview of being able [00:37:00] to fix things to make things better.
It’s both a blessing and a curse. And I think scientists in general suffer from this blessing and curse because we see the world around us and things we would like to change to make it better. And then we sometimes think, am I doing the right thing? Or if what I’m doing, can I change what I’m doing right now to add more variety or validity to what I’m doing to make it even better?
Um, it is enabling us as a scientist or an engineer can be enabled to make small changes to their career to make their career more in line with what they want to do. Um, I grew up in a restaurant. And in a restaurant, we can change the recipe of something to enable it to taste different or better, or, but we also run the risk of annoying our customers.
That’s true in science and engineering as well, but customers [00:38:00] are, are a little less, um, unhappy if you tell them it lasts longer, it’s cheaper to make. And I mean, if you say that about a hamburger, your hamburger lasts longer and it’s cheaper to make. People may not be quite as happy. So I’m glad I’m in engineering and not in food science.
I’m glad I’m in. Yeah. Yeah. That’s a, that’s a very good point. Uh, we, it’s a, it’s a curse and a boon and a curse. Uh, at the same time, there’s like, yeah, this is definitely something that scientists have and scientists and engineers have in common. Um, so Dumos from your decades of experience, um, If you had three wishes to improve the, the world, so to say, or the world that you operate in the, the engineering aspects of things or the scientific aspects of things in the different industries as well, from space travel to solar to electric mobility, uh, so on and so forth.
What would you ask for like three wishes to improve the [00:39:00] field? Uh, and I’m not promising anything here. Okay. Just want to know you. Well, I saw the the question earlier from you and at first I had a very simple answer improve funding for basic research. But then I thought that’s that’s great. But that’s how that happens.
And there is even more funding for climate research there. There is. But here’s where it may not be where I would adjust that answer where you see the effects of climate change the most on the people that are the least able to afford to fix it and where we see the least amount of money spent. Because there’s no money to be made from fixing the dams in Libya that broke after Hurricane or Medicaid, Daniel came in and destroyed so many lives with that, that terrible infrastructure situation.
Why was that infrastructure even there? Why couldn’t have it have been changed for [00:40:00] something else? How can we change how we design our cities and our power grids and our ability to support people, especially in places where People do not have the money or the resources to develop that. And I think, right now, there was some funding that had occurred, and this was just in our newspaper, there’s an attempt to restart the funding for AIDS prevention in Africa.
And so, um, back in 2007, I believe, or I think, or it may have been 2004, actually, I think it started in 2004, there’s a huge, A grant of money sent from the U. S. to support AIDS, uh, AIDS, um, drug research and then AIDS drug delivery in Africa. This was to a place where there was no economic benefit to the United States for this.
At least no immediate economic benefit. And that it was only a humanitarian benefit. And yet the result of that was 25 million lives were saved. [00:41:00] By that, by that type of work. However, now there’s a question of if that will even continue and many people will ask, well, what does it matter to me? That is of no economic benefit to me.
Why should I save 25 million lives in a country? I don’t know, or I will never visit, but that’s I think the kind of thinking. So if I had a magic wand, I would say I would like to see that kind of forward thinking applied in climate scientists and material science. Thanks. In electric and hybrid vehicles and power distribution, because I think one of the biggest problems we have is those parts of the world where we could improve people’s outcomes are also the parts of the world where we could get the most solar, the parts of the world where we could generate the energy to make the rest of the world a better place.
There’s a lovely podcast called hardware to save the planet. And there’s a scientist from MIT who had reimagined and he had worked at JPL to think about [00:42:00] how energy could be used, how energy could be transferred around the world. And he had a fascinating idea. The most energy dense item in the world, which is the most abundant thing in the Earth’s crust.
Is aluminum or bauxite and the, and the processing of aluminum is 10 megawatts per kilogram. Take a 10 megawatt hours per kilogram. If you take aluminum powder and you start a redox reaction, you will have an enormous explosion. Why? Because there’s a lot of energy in aluminum and when aluminum reacts with water, you get hydrogen gas.
Think about the fact that we could take something like hydrogen gas, we could have solar farms, generate, make aluminum with bauxite from the earth’s crust and ship out alum, uh, just, uh, and so you create a smelt aluminum right there on site and then ship aluminum and the aluminum is your energy [00:43:00] storage device.
It is not a piece of equipment for building something with. It is a battery. A solid battery. Yeah, no one imagines aluminum when we are talking about batteries. No one. Aluminum is the insulator. Aluminum is the lightweight metal. No one, no one associates aluminum with battery or generating electricity, so to say.
But this is Yeah, for sure. So funding, forward thinking. I think that’s a very good point with the forward thinking. I think this is very, very, very important. So like long term thinking, not just like, okay, what am I getting tomorrow with this experiment? What am I getting day after tomorrow? Am I getting a benefit out of it?
And so on and so forth. That’s very, very important. You have a third wish if you want to put it out, uh, in the, in the world. I think the third wish is to, [00:44:00] once again, raise the, create the, a good relationship between non scientists and scientists. Right now, I think we’re suffering in this world with a unhappiness with the elite, in the U.
S. at least. There’s a culture war with this, and the culture war is that people who are elite or scientists Um, are not on the side of the working person of the person who has to survive day by day. And I think that relationship and podcasts like these are a way to start that communication. And to say, here we are as scientists, concerned scientists, thinking about ways to make the world better and to distribute funds in a way that’s forward thinking.
No, it doesn’t make me money right now, but I know in 20 years, the world will be a better place because I spent money there. So, so that making, making friends. [00:45:00] That’s it. I just want to make friends making friends. Yeah. That’s a, that’s a very, very, uh, valid wish. And I am going to steal that one from you as well.
Demos. This has been so, so, so amazing. This has been, we are well over the time limit, but I. Feel like I can talk to you for hours, but before I let you go, uh, what can the followers of the Real Scientist Nano Twitter account expect in the week that you’re taking over the Twitter or X account, the Real Scientist Nano, which is part of like the second part of the, under the microscope.
So for me, one of the things I’m going to do is try to give people a little bit of knowledge of what this. Revolution in electronics is about because we’re silicon carbide and wide band gap is now a part of our daily life. And so few people know about it. And what’s happened is, [00:46:00] is replacing silicon. We always think of silicon as like, what we make transistors out of, but now, we’re making transistors out of other materials.
These materials are completely different. And are much more in line with what gold is. And not what I mean, I’m not that cool, but what diamond is so, so these, these materials are more like jewelry. They’re not just silicon. Silicon is sand. So, um, silicon carbide, carbide, carbon compounds, diamond transistors.
Now we’re talking about. You know, mind blowing stuff, how, what do you mean? You can take my jewelry and turn it into an electric vehicle power generator. That’s essentially what we’re talking about. Of course, Ruby has been used in lasers for a very long time. So, so we know that, that minerals do some pretty amazing things.
So we’re going to talk about, um, wide band gap technologies and how they’ve been used. They’re going to make the world a better place in, in, in some ways, though, that’s not, that’s, [00:47:00] that’s a very narrow area and it might be boring for some people. So I’ll see if I can throw in some other material science stuff that might, um, also engage people in other areas, but LEDs, for example, the things we used to light up our homes is part of this technology.
Uh, these alum, uh, gallium Maronite, for example, is what’s used to make an LED. So that is, again, a wideband gap electronic that we use every day and has enabled better living. Mm-Hmm, . Yeah. And, and we also want pictures of the glowy tubes and Oh, yes. The tridium, the blue dot, uh, no, what was it? Blue Planet?
The, the Space Travel Company of, uh Oh yeah. Blue Origin. Yeah. Origin, yeah, we want to, we want to know what happened there. What did you do? Whatever you can share. I’m going to offer this. I think I might change and maybe we’ll just use the topics from today’s conversation. [00:48:00] We’ll just touch on each of those this week because there’s something Blue Origin in.
I want to throw this in that I was very impressed with and I didn’t know about. This was a black program, so even, even the senior engineers didn’t know about this, a quantity of lunar rock was brought back to earth. Some of that RO rock was given to Blue Origin scientists, not, not me, other scientists who are really much smarter than me, much smarter than me.
They crushed the rock, melted it, and made. And made solar cells out of lunar rock samples. And how did they perform? How did, how did, yeah, okay. They converted light into electricity exactly the way they’re supposed to. What was the material? What was the composition? What was the, like, what was the topography brittle?
Was it crystalline? I will, I will give you all of that detail in, in the links, but yeah, there’s some good papers published about that. Oh [00:49:00] my God. I feel like you should be like tweeting from the real scientist nano account, like every second week or something, if not every week. And I need to have you back on the podcast on under the microscope to get into, we didn’t even get to speak about the diamonds in transistors.
Yeah. There is just so much that, uh, I can tap into. And before we close, I want to give you the stage. And so where can people find you other than power america. org? Where, where can people find you? Where do you want people to, where do you want to be, want to be found? Well, um, my wife and I have a podcast called Luxi.
org, uh, at Luxi, and it is a science of luxury items podcast. We’ve been doing that for a couple of years. That is an area where you can find us. The other place, and I, and, and we’ll post links to that. The other places on my LinkedIn page, um, I don’t engage with social media as much as I [00:50:00] should. And it’s partly because I’m, I’m just, I will change my evil ways, I know, I hope to, I hope to change my evil ways and get engaged with Instagram more.
I’m not on Instagram, I follow Instagram, I’m not really reading a lot about it, so I’m working on it. There are things that I do well and things I don’t do well, and social media is one of those things that I’m hoping to do better. But, um, you don’t have to be on social media and your lovely wife, uh, Alexis, uh, is doing a fantastic job on Instagram with look science.
Everyone check out the look science podcast because this is super interesting, super fascinating. And it’s also a lot of fun, to be honest, you learn something new. with every episode, literally. And I pour a lot of energy into Luxai. So that’s, so what I don’t pour into social media is in the audio editing and production of, of those, of those podcasts.And this has been [00:51:00] lovely and looking forward to having you on Real Scientist now.
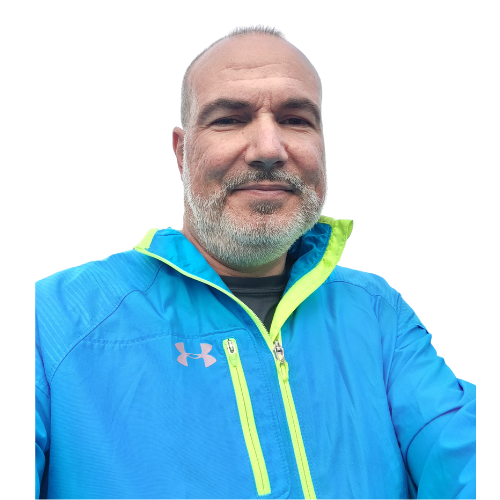
Space Travel, Heavy Ions, Electric Cars
Dimos is the Director of Power Electronics at Power America (USA).